Choosing an option
Two major considerations for teachers In selecting the one option (of the five
available) for their students to study, are first their students' likely interest in the topic and
secondly their ability to cope with
the amount and complexity of the material. Other considerations are likely to be
how well the material integrates with and reinforces core material and how
straight-forward and/or predictable are likely examination questions on the
material. The availability of equipment for laboratory work is another
consideration.
Judging by the number of pages I needed to cover
the material in each of the three options treated in Conquering Chemistry,
the Shipwrecks, Corrosion and Conservation option appears to be the shortest, and the
material does to a significant extent reinforce and extend core material, though
some of it can be difficult to understand for weaker students. In some ways the
material in Industrial chemistry may be more straight forward, but apart
from chemical equilibrium it does not flow as directly from core material. There appears to be much
more material in Forensic chemistry and much of it is quite complex for
students whose background in organic chemistry is just the core material. While
a good option for students with a strong biological interest, it could be
daunting for weaker students.
With the current heavy emphasis on factual recall
in exam questions, the quantity of material to be assimilated is probably a key
consideration in selecting an option.
In writing Conquering Chemistry I thought
that the other two options, Biochemistry of movement and Chemistry of
art, contained too much material of too diverse a nature for the seven weeks
available for the study of the option. Of course these options will particularly
appeal to certain groups of students – those strongly interested in sport and
dance and those with a strong artistic bent – and strong motivation can easily
overcome barriers that may seem daunting to others.
Some
teaching points
1.
Exam questions
HSC exam questions in
the Options section of the paper differ somewhat from questions in the core
section in that .
· |
there is more
emphasis on recall of learned information about the context than on the
underlying chemical principles |
· |
there is always
at least one question about a compulsory experiments, often with a bit
about risk assessment |
· |
there is always
at least one extended response question (question with five or more
marks) and it usually is of the discuss, analyse, assess, evaluate type,
and |
· |
apart from the
Industrial Chemistry option there is rarely a problem-solving or
calculation question. |
Because of this absence of calculations and
problems from the options sections of the HSC exam paper, there will be no
Further Exercises sections on the Options pages (though a few exercises will be
interspersed through the text).
2.
Electrolysis
The syllabus requires students to describe what
happens at the anode and cathode 'during electrolysis of selected aqueous
solutions'. Its hard to know just what aqueous solutions are required, so to be
on the safe side CCHSC treats the whole range of aqueous solutions (see pages
407-11). Note that it is necessary to study pages 347-51 in Option 1 – they
are also part of Option 2 (except for molten sodium chloride). The results of
electrolysing aqueous electrolyte solutions can be summarised as follows:
Different possibilities for
electrolysis of aqueous solutions of ionic substances:
|
At
the cathode (–ve) |
At
the anode (+ve) |
Example |
(a) |
the cation is reduced |
the
anion is oxidised |
CuCl2 |
(b) |
the cation is
reduced |
water
(or OH–) is oxidised (to O2) |
CuSO4,
AgNO3 |
(c) |
water (or H+) is
reduced
(to H2) |
the
anion is oxidised |
KI,
KBr |
(d) |
water
(or H+) is reduced
(to H2) |
water
(or OH–) is oxidised (to O2) |
Na2SO4, H2SO4, NaOH |
All of the above are with inert electrodes –
electrodes that do not undergo chemical change during the electrolysis. Common
cases in which an electrode reacts are electrolysis of aqueous solutions of
copper and silver salts using a copper or silver anode respectively (Cu
®
Cu2+ and Ag ®
Ag+).
Most electrolyses are
not particularly sensitive to the concentration of the ions present, the
exception being chloride where concentrated solutions produce chlorine gas
while dilute ones form hydroxide (page 350).
Correlation
with electrode potentials
What happens during
electrolysis of aqueous solutions can be roughly correlated with electrode
potentials (pages 410-11). Basically, the higher the electrode potential the
more readily the reduction half reaction occurs and the less readily the
oxidation (reverse) half reaction occurs. Hence Ag+ and Cu2+
are more easily reduced than is H+, but H+ is reduced in
preference to Al3+ or Na+ (Eos for Ag,
Cu, H, Al, Na are
+0.80, +0.34, 0.00, –1.66 and –2.71 V respectively). Iodide is more easily
oxidised than bromide which in turn is more easily oxidised than water; however
water is more easily oxidised than is fluoride (Eos for I, Br,
Cl and F are +0.54,
+1.09, +1.23, and +2.87 V respectively). Water and Cl are close together
(1.36 and 1.23 V) so changing the concentration of chloride can change which is
oxidised preferentially. (Remember Eos refer to
reduction half reactions.)
Stating things less
precisely: at the cathode the species with the algebraically higher Eo
gets reduced (H+ rather than Mg2+) while at the anode the
species with the lower Eo gets oxidised (I–
rather than Cl–)
Exercise
1. |
Why
is it sometimes said (as in the HSC Syllabus document) that Galvani,
Volta, Davy
and Faraday contributed to increasing understanding of electron-transfer
reactions, when in fact electrons were not known in their time, nor was
the nature of chemical reaction or the concept of chemical equations fully
developed? |
3. Crevice corrosion
Crevice corrosion is
corrosion that develops in microscopic gaps between sheets of metal that are
held tightly together by physical means, such as by rivets or by nuts and bolts
(and washers). It can be more severe than ordinary surface corrosion. When
overlapping sheets are immersed in salt water, Figure 1, there will be a thin
film of conducting water between the sheets, so normal corrosion will occur. But
soon all the oxygen in the gap (crevice) will be used up. Then Fe tends to
oxidise to Fe2+ in the crevice while oxygen gets reduced to OH–
on the exposed surface. Some of the Fe2+ diffuses out of the crevice
and meets with the OH– and so rust builds up around the exposed
parts of the joint. If there is chloride in the film of water, it can form
insoluble hydroxy chloride with the Fe2+ and this makes the water in
the crevice slightly acidic. This more acidic environment can accelerate the
oxidation of Fe and so accelerate rusting. There will be a build up of rust
around the exposed edge of the joint, and in the crevice there will be some
normal rust formed (because oxygen does slowly diffuse into it), there will be
some deposits of insoluble hydroxy chlorides (see CCHSC page 434), but
more importantly there will be considerable pitting of the iron surfaces which
can lead to a weakening of the joint.
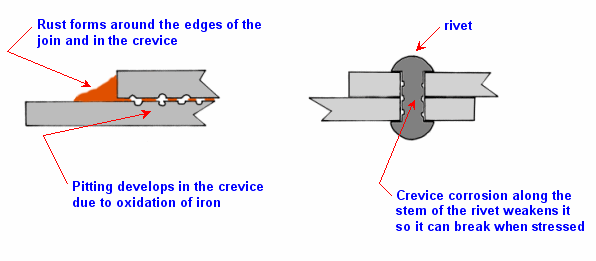
Figure 1 Crevice corrosion and its effect
on rivets
Crevice corrosion is of
particular concern when sheets of metal are riveted together, because by eating
away the stem of the rivet, it can weaken the rivet and cause it to break. The
best way of overcoming crevice corrosion is to avoid such overlapping joints
(that is to eliminate crevices). This is why welding (CCHSC page 401) is
preferred to riveting.
4.
The Titanic
Interest in the Titanic
and its dramatic sinking increased significantly when the wreck was
discovered in 4 km deep water in 1985 and when in the following decade many
artefacts and as well part of the hull were recovered from the ocean floor.
Locally further interest was aroused by the opening of an extensive display
about this ship by the Australian Maritime Museum in the late 1990s. The
production of a very popular Hollywood blockbuster movie in 1997 also helped.
Titanic sunk because it
hit an iceberg that tore a huge hole in its hull just below the waterline. The
reasons commonly given for the hull fracturing are (1) compared with present-day
steels, its steel contained more carbon and small but significant amounts of
sulfur and these made it more brittle and hence more likely to fracture rather
than just bend and dent, and (2) the steel plates were riveted together (rather
than being welded as happens today) and had suffered crevice corrosion which had
weaken the rivets so that they broke when stressed by the collision; there had
been a longer than usual time between launching the hull and completing its
fit-out before the maiden voyage and this could have aggravated corrosion.
The steel and riveting
in the Titanic were in no way inferior to those used in other ships of that era.
It was just bad luck that such a big ship hit such a large iceberg, though
perhaps it was travelling faster than was prudent in such conditions; the ship
was trying to set a new cross-Atlantic time record.
Finding that the wreck
of the Titanic was severely corroded when only limited corrosion had been
expected led to the discovery of bacterial corrosion (CCHSC page
430).
Concentration
and solubility of gases in sea water
The solubility of a gas in water is the maximum concentration of that gas
in water when the water is in contact with the gas. The solubility of a gas is
proportional to the pressure of the gas above the solution and it increases as
the temperature of the solution decreases (CCHSC pages 426-7). For oxygen
gas
S = kP
where S is solubility, P is the pressure of oxygen gas above
the solution and k is the proportionality constant. k = 2.0 x 10–5
mol L–1 kPa–1 so for an oxygen pressure of 100 kPa the
solubility is 2.0 x 10–3 mol L–1.
However the concentration of a gas in water can be significantly less
than the solubility if the solution is not in continuous contact with the gas.
This is the situation that applies in deep sea water. The concentration of
oxygen is much less than the solubility.
Exercise
2 (a) |
The gas from a
cylinder of oxygen was slowly released into sea water near the surface
where the atmospheric pressure was 100 kPa and the temperature 20oC; the
water became saturated with oxygen. A sample of the water was taken from
near the cylinder soon after all bubbles of gas had disappeared and the
oxygen concentration measured. It was found to be 1.4 x 10–3
mol/L. Explain why this value is different from
(i) the one given in Table
12.1 on page 427 (2.8 x 10–4 mol/L) and
(ii) the one calculated in the previous section. |
(b) |
The gas from
another cylinder of oxygen was slowly released into sea water at a depth
of 70 m where the ambient pressure was 800 kPa and the temperature 4oC;
again the water near the cylinder became saturated with oxygen . A
sample of this water was taken soon after all bubbles of gas had
disappeared and the oxygen concentration measured. It was found to be
close to the value expected from the equation in the previous section. What is
this value? |
(c) |
A sample of the
water at 70 m depth was taken just before the oxygen had been released
from the cylinder in (b) and it was found to contain oxygen at a
concentration of 1.1 x 10–4 mol/L. Why is this value so
different from the one in (b)? |
5.
Metals for shipbuilding
Twice in recent years
(2004 and 2007) the HSC exam paper has had extended-response questions based
upon developments in technology influencing choice of metals for shipbuilding.
Actually over the past one hundred years or so there have not been any dramatic
changes in the metals used for shipbuilding – only incremental changes such as
improved steels. In recent years there has been a trend towards aluminium and
its alloys for smaller vessels such as ferries and catamarans and leisure craft,
but large ocean-going cargo and passenger ships still have steel hulls. The
account in CCHSC pages 401-4 should be adequate for all HSC purposes.
6.
Metals in contact
The key point to
remember here is that when two metals are in contact, the one with the
algebraically lower (smaller) electrode potential will oxidise preferentially,
so magnesium will oxidise instead of iron, iron will oxidise instead of tin.
The common situations
where corrosion of metals in contact arises are
· copper
water pipes connected to steel pipes
· tin cans
(steel coated with tin)
· galvanising
· cathodic
protection of ship hulls and underground pipelines (using sacrificial
anodes).
Note however that the
two metals must be in physical contact (or at least connected by a conducting
wire) for this preferential oxidation to occur. If instead the two metals are
placed in the one electrolyte solution (as in the 2005 HSC exam paper), then
each corrodes or reacts independently of the other.
In that 2005 exam
question students were asked to predict what would happen if pieces of magnesium
and iron not in physical contact were placed in the one electrolyte solution
that contained both Mg2+ and Fe2+ ions. The Mg metal would
react with the Fe2+ ions
Mg(s) + Fe2+(aq) ®
Mg2+(aq) + Fe(s)
The magnesium would become covered with a blackish deposit of iron as the
magnesium itself slowly dissolved.
In addition the piece of iron would rust – develop a brownish coating of
hydrated iron(III) oxide:
Fe(s) ®
Fe2+(aq) + 2e–
O2(aq) + 2H2O(l) + 4e–
®
4OH–(aq)
Fe2+(aq) + 2OH–(aq) ®
Fe(OH)2(s) ... ®
Fe2O3.xH2O(s)
An excellent example of
corrosion of metals in contact was the Statue of Liberty in New York, USA (see
below).
Exercise
3. |
Bolting
blocks of zinc or magnesium to the hulls of ships protects them from
corrosion. Would bolting a block of zinc or magnesium to the body of a
motor car protect it from rusting? Explain why or why not.
|
7.
Acidic and alkaline forms
of equations
Sometimes equations, particularly half equations, are written in terms of H+
ions and at others in terms of OH– ions; for example
2H+ + 2e–
® H2 (acidic form)
2H2O + 2e–
®
H2 + 2OH– (alkaline form)
We generally write the form that is most applicable to the solutions being
discussed – whether they are acidic or alkaline.
An 'acidic' form of an equation can be converted to an 'alkaline' form as
follows:
1.
|
To
each side of the equation add a number of OH–s equal to the
number of H+s present |
2.
|
On the side that has both OH–s and H+s, combine these
into H2O
|
3.
|
Cancel out any molecules of water that appear on
both sides of the equation
|
For example:
|
|
2H+ + 2e–
® H2
2H+ + 2OH– + 2e–
® H2 + 2OH–
|
giving
|
|
2H2O + 2e–
®
H2 + 2OH– (no H2O to cancel)
|
Similarly for the production of oxygen in the
'electrolysis of water'
2H2O
® O2 + 4H+ + 4e– (acidic form)
Add 4OH– to both sides: |
2H2O + 4OH–
® O2 + 4H+ + 4e– + 4OH– |
Combine to 4H2O:
|
2H2O + 4OH–
® O2 + 4H2O + 4e–
|
Cancel 2H2O from each side to give:
|
4OH–
® O2 + 2H2O + 4e– (alkaline form)
|
To convert an alkaline form to an acidic form add H+s to both
sides and proceed similarly.
Electrolysis of
water
Such conversions are often required in writing equations for
so-called electrolysis of water experiments.
For the electrolysis of dilute sulfuric acid (hydrolysis
of water) we would use acidic forms:
At the cathode: 2H+ + 2e–
® H2
At the anode: 2H2O
® O2 + 4H+ + 4e–
Overall (double the first and add to the second): 4H+ + 2H2O
® O2 + 4H+ + 2H2
Cancelling out 4H+ gives: 2H2O
® 2H2 + O2
For electrolysis of sodium hydroxide solution
(also hydrolysis of water) we would use alkaline forms:
At the cathode: 2H2O + 2e–
® H2 + 2OH–
At the anode: 4OH–
® O2 + 2H2O + 4e–
Overall: 2H2O
® 2H2 + O2 (double the 1st, add to 2nd, cancel out
2H2O + 4OH–)
For electrolysis of near neutral solutions of
salts such as sodium nitrate, magnesium sulfate or potassium fluoride which
are also electrolysis of water we could use either the acidic pair or the
alkaline pair (but don't use one of each!).
Oxidation by O2 in
corrosion contexts
In corrosion contexts
oxidation is often by oxygen gas either dissolved in water or from the air.
Again we can write the reduction half equation in either acidic or alkaline
forms:
O2 + 4H+ + 4e– ®
2H2O
O2 + 2H2O + 4e–
® 4OH–
8. Different
Eos
for different forms of similar half reactions
From a table of standard
electrode potentials we find that the two forms of what is essentially the one
process have different Eos: for example
(i) |
O2 + 4H+ + 4e–
® 2H2O |
Eo
= 1.23 V |
(ii) |
O2 + 2H2O + 4e–
® 4OH– |
Eo
= 0.40 V |
The reason lies in the 'standard' part of the name. The standard electrode
potential is the value when all solutes in the reaction (equation) are present
at concentrations of 1.00 mol/L (and gases present at partial pressures of 1.00
atmosphere). So the value for equation (i) is for [H+] = 1.00 mol/L
(and so with [OH–] = 1.0 x 10–14 mol/L).
The Eo value for equation (ii) is for [OH–] = 1.0
mol/L and so [H+] = 1.0 x 10–14 mol/L).
We can
calculate a (non-standard) electrode potential for both of these half reactions
for [H+] = [OH–] = 1.0 x 10–7 mol/L (that
is pH = 7.0; neutral solution). It is 0.82 V for both of them.
|
O2 + 4H+ + 4e–
® 2H2O |
EpH=7.0
= 0.82 V |
|
O2 + 2H2O + 4e–
® 4OH– |
EpH=7.0
= 0.82 V |
Getting the same value
for both should be no surprise: after all both equations simply represent the
reduction of oxygen gas in water. Or looked at from the other direction, they
both represent the oxidation of water to oxygen gas.
Similarly for
|
2H+ + 2e–
® H2 |
Eo
= 0.00 V |
|
2H2O + 2e–
® H2 + 2OH– |
Eo =
–0.826 V |
the first value refers to [H+] = 1.00 mol/L while for the second [OH–]
= 1.0 mol/L. Again we can calculate a value for pH = 7.0
|
2H+ + 2e–
® H2 |
EpH=7.0
= –0.41 V |
|
2H2O + 2e–
® H2 + 2OH– |
EpH=7.0
= –0.41 V |
Again the same value should be no
surprise because both half equations just represent the reduction of water to
hydrogen gas.
(For the benefit of teachers:
these calculations are using the Nernst equation which you would have met in
first year university chemistry. Check it out in any standard first year
university textbook.)
Remember, it does not matter
whether we write the equations as above or with one electron only: H+
+ e–
® ½H2 . The Eo
value is the same (see CCHSC page 59).
9.
Australian maritime archaeological projects
The syllabus requires
students to gather information from secondary sources to compare conservation
and restoration techniques applied in two Australian maritime archaeological
projects. The best known projects are:
·
|
Salvage and
restoration of six cannons and several blocks of iron ballast from the
Endeavour (off Cooktown) in the 1970s and 80s (CCHSC
page 438) |
·
|
Salvage of
artefacts (including cannons) from the Pandora (off Torres Strait) in
the 1990s and still going on (just mentioned in CCHSC page 392,
but similar to techniques of pages 434-40) |
·
|
Salvage and
restoration of part of the Dutch ship Batavia and many artefacts that it
contained off the coast of Western Australia (1970s and 80s) (CCHSC
pages 439-40) |
·
|
Salvage and
restoration of silver and copper coins from various Dutch ships off the
Western Australian coast in the 1980s and 90s. (CCHSC pages
436-7) |
Cannons
from Cook's Endeavour (CCHSC page 438)
The six cannons that Cook jettisoned were located by scientists from the Philadelphia
Institute (USA) in 1969. They recovered some of them; an Australian group
recovered the rest in 1970 along with about 90 iron ingots (used as ballast in
the ship). The cannons were restored by what was then called the Australian
Defence Science Services of the (Commonwealth) Department of Supply. Of the
cannons, one went to the Philadelphia Institute, one to the Greenwich Maritime
Museum (in Great Britain), one to the National Museum of New Zealand; of the
three that stayed in Australia, one is at Kurnell, one at the Cooktown Museum
and the other at the National Maritime Museum in Sydney.
An excellent account of the recovery and restoration of these cannons, along
with many informative and attractive photos, is in the book,
Cook's Cannon and Anchor, Dennis Callegari, Kangaroo Press, Sydney 1994.
A more technical and detailed account of restoration of objects recovered
from the sea is the book,
Conservation of Marine Archeological Objects, Colin Pearson (ed),
Butterworths, 1987.
This book discusses restoration of wooden and leather objects as well as metal
ones.
The Pandora
Other shipwrecks that have aroused considerable Australian interest are those
of the Bounty and the Pandora. After the mutiny of the Bounty in 1789
and Captain Bligh's epic journey back to England, the British navy sent the Pandora
to capture the mutineers and recover the Bounty. Some mutineers were
captured in Tahiti but the Bounty was not found (It had been sailed to
Pitcairn Island where it was burnt.) On the return journey the Pandora struck
the Great Barrier Reef off Cape York and sank. This wreck has been
located in recent decades and objects are being salvaged and restored from it (see the photo of the Pandora cannon on page
392-3).
The Batavia
The Batavia, a ship of the Dutch East India Company that was involved in trade
between the Dutch East Indies (now Indonesia) and Holland, was wrecked off the
Western Australian coast in 1629. The wreck was discovered in 1969 and between
1972 and 1976 many artefacts and much of the hull were excavated, salvaged and
restored. The timbers from the hull were painstakingly and slowly restored and
reassembled. The rebuilt sections of the hull are displayed in the Maritime
Museum in Fremantle while restored artefacts are displayed in that museum
and in the Western Australian Museum in Geraldton.
Exercise
4.
|
Silver
coins recovered from long-sunken shipwrecks need to be treated
electrolytically (as described on pages 422–3) in order to restore them
to as closely to their original condition as possible. However gold coins
recovered from such wrecks can be restored either by chemically dissolving
away any deposits or by mechanically removing them (by chipping or by
using a gentle abrasive). Why is electrolysis not necessary or effective
for restoring gold coins?
|
10. Restoration of
salvaged wood?
There is no specific mention of restoring wooden objects in the current syllabus
document, so it was surprising to see a question on restoring a wooden chest in
the 2006 exam paper. Fortunately there is sufficient information on restoring
wood in CCHSC pages 439-40 to answer this question.
11.
Websites
Unfortunately the
addresses of all three websites listed on CCHSC page 441 have changed.
They now are:
http://www.conservationsolution.com/projects/about-projects (Click on
Archaeological Elements and Antiquities or Objects and select items about Titanic)
http://www.museum.wa.gov.au/collections/maritime/march/Batavia/batavia.html
http://www.mq.qld.gov.au/exhibitions/pandoragallery.asp
Supplementary material
1.
The Statue of Liberty (metals in contact)
The Statue of Liberty is a 46 m
high statue of a woman holding aloft a flaming torch. It stands on a small
island near the entrance to New York harbour. Liberty was a gift from France to
commemorate the France-US alliance during the American war of independence. It
was built in France and transported in pieces to New York where it was assembled
and mounted on a large pedestal. It was finally completed in 1886.
The statue was made with an
internal steel support structure (skeleton) with a copper 'skin' that took on
the characteristic bluish-green colour of air-oxidised copper – a mixture that
includes copper hydroxide and carbonate called a patina. The patina is not only
attractive in appearance but also it protects the underlying copper from further
corrosion because it forms an impervious layer.
The people who made the statue
knew about corrosion of metals in contact and tried to avoid this corrosion
problem by using strips of asbestos coated with shellac to insulate the steel
supporting bars from the copper skin. However over time (about 100 years),
particularly with movement of the copper skin relative to the steel supports
(due to copper's greater thermal expansion than steel), this insulation was worn
away and severe corrosion set in. The steel supports rusted with the supporting
tips of many of them breaking off so that the copper skin was able to flap about
in the wind and tear and become damaged.
Finally in 1984-86 the statue
underwent a major restoration. The rusted steel bars were replaced with
stainless steel ones (to resist corrosion) and as added protection teflon
sleeves were used to insulate the copper skin from the stainless steel supports.
These sleeves also allowed the copper skin to move more easily with expansion.
Apart from repairing some minor damage, the copper skin with its lovely patina
was preserved.
Today a greater threat to the
statue is acid rain. It slowly dissolves the protective patina (H+ +
Cu(OH)2 and CuCO3) and exposes the thin copper skin to
further corrosion.
2.
Acid sulfate soils (and
acid mine drainage)
On page 430 it is explained how
certain bacteria can reduce sulfate (to sulfide) and so bring about corrosion of
iron at ocean depths too great for there to be sufficient dissolved oxygen to
corrode the iron. This may bring to mind an environmental problem that often
occurs in Australia, namely acid sulfate soils (which is not a very
informative name!).
Some soils when they are
disturbed – dug up for farming or excavated for sub-divisions and buildings
– produce acid which drains into waterways and so has a detrimental effect
upon aquatic life. The problem here is oxidation of sulfide to sulfate – in
some ways the opposite to the bacterial oxidation of shipwrecks.
The soils that produce acidic
run-offs are ones that contain iron pyrites, FeS2. This unexpected
formula arises because the two S atoms are covalently bonded together to form
the S22– ion: –S–S–. The
peroxide anion O22– in Na2O2 or
BaO2 is similar: compare with the structure of hydrogen peroxide
H–O–O–H .
On exposure to air (oxygen) iron
pyrites oxidises to Fe(II) sulfate and sulfuric acid:
2FeS2(s) + 7O2(g) + 2H2O(l)
® 2Fe2+(aq) + 4SO42–(aq)
+ 4H+(aq)
(the products could be written 2FeSO4 + 2H2SO4)
This overall equation is made up of the two half equations:
FeS2 + 8H2O
® Fe2+ + 2SO42–
+ 16H+ + 14e–
O2 + 4H+ + 4e–
® 2H2O
The Fe2+ is then oxidised to Fe3+
(at the low pH involved, generally by bacteria such as Thiobaccillus
ferrooxidans) and precipitates out as a yellow-brown gelatinous Fe(OH)3.
This leads to further acid production:
4Fe2+(aq)
+ O2(g) + 4H+(aq)
® 4Fe3+(aq) + 2H2O(l)
4Fe3+(aq) + 12H2O(l)
® 4Fe(OH)3(s) + 12H+(aq)
Water draining from coal mines is often quite
acidic. The cause is this same series of reactions: mining exposes iron pyrites
to air and so oxidation occurs. This is called acid mine drainage and is
a serious problem for most coal mines.
3. Anodisation of aluminium
On page 406 it was stated that
aluminium was a passivating metal, meaning that it readily forms a protective
oxide layer that prevents further oxidation (corrosion). This protective oxide
layer can be made thicker and hence more effective in protecting the underlying
metal by a process called anodising.
The aluminium object to be
protected is made the anode in an electrolysis cell: the electrolyte is a
solution of sulfuric acid and the cathode a piece of unreactive metal (for
example, stainless steel or platinum). Under these conditions the process that
occurs at the anode is
2Al + 3H2O
® Al2O3
+ 6H+ + 6e–
This thickens the oxide layer and so make the aluminium more resistant to
corrosion.
If certain dyes (coloured
materials) are also present in the electrolyte, they can become incorporated in
the oxide layer and so give it a definite colour. In this way aluminium objects
can be given distinctive and durable colours. Gold and bronze coloured anodised
aluminium objects, such as car and door keys, are fairly common. Less
fashionable now than they were 20 years ago, brightly coloured saucepan lids and
cake containers can probably still be seen in your grandmother's kitchen.
Answers
to selected HSC Exam questions
2007 Question 29(b)
Corrosion is a major problem for
vessels that have to operate in a variety of aquatic environments
Analyse how the factors in aquatic
environments have impacted on the choice of metals used in the construction of
vessels over time.
6 marks
(Transcribed from the 2007
Board of Studies HSC Chemistry examination paper)
Comment |
Sample answer |
Analyse in this context
means identify the factors and explain how they have
impacted ...
Note the variety of aquatic environments in the question, so our
vessels do not just operate in sea water.
There are 6 marks for this question so you have 11 minutes to answer it
and need at least six key facts.
The three factors are water, oxygen and salt. State this (even if the
water seems self-evident).
These three factors lead to corrosion of the metal so ideally we should
choose a metal that does not corrode. Explain what corrosion is. However
the main factor affecting the choice of metal has been cost. Generally
it has been more cost-effective to protect steel against corrosion than
to use a corrosion-resistant metal.
You should mention that the corrosive nature of aquatic environments did
lead to the use of aluminium in some vessels and that the development of
stainless steels has resulted in their use in parts of big ships.
You could include two, three or all four of the following equations if
you can recall them quickly.
Fe ®
Fe2+ + 2e–
O2 + 2H2O + 4e–
® 4OH–
Fe2+ + 2OH–
® Fe(OH)2(s)
Fe(OH)2 + O2
® Fe2O3.xH2O(s)
(rust) |
The factors that impact upon
the choice of metals used are the actual watery nature of an aquatic
environment, the presence of dissolved oxygen in the water, and in
oceans the presence of dissolved salt as well. These lead to corrosion
of many metals and alloys.
Steel was the first metal (alloy) used for making vessels (ships),
because it was readily available at a reasonable price. Unfortunately
iron (steel) rusts (corrodes). For rusting to occur there needs to be
oxygen and water present. Rusting is speeded up if there is salt (sodium
chloride, NaCl) dissolved in the water. Rust is hydrated iron(III)
oxide. Since vessels operate in the surface layers of the water body
they are in, there are always water and dissolved oxygen present, so
steel needs to be protected either by paint or by using sacrificial
electrodes.
It would be better to use some metal or alloy other than iron that did
not corrode but until recently none was available at a competitive
price, even after taking corrosion protection into account. The main
factor in the choice of metal for making ships has been cost.
In recent decades aluminium and various alloys of it have become more
readily available and have been used for making smaller vessels such as
pleasure craft and catamarans and for various fittings and
superstructures on large ships (though not for hulls, mainly because
they lack the required structural strength).
In the century after steel-hulled ships were first built there were
steady improvements in the composition of steels used (less carbon, near
zero sulfur and phosphorus) that increased their rust resistance.
Changes from riveting to welding for joining sheets of steel also
improved rust resistance (by avoiding crevice corrosion).
Development of stainless steels that do not rust led to their use in
many fittings in ships though stainless steel is still too expensive for
hulls. |
2008 Question 30(d)
Several marine archaeological projects exist around Australia. Compare the
conservation and restoration techniques used in TWO of these projects with
reference to the chemistry applied.
7 marks
(Transcribed from the 2008
Board of Studies HSC Chemistry examination paper)
Comment |
Sample answer |
The syllabus requires
students to gather information from secondary sources to compare conservation
and restoration techniques applied in two Australian maritime archaeological
projects. There is an implication in this question that the answer should refer to current projects which is not a
restriction incorporated in the syllabus. The difficulty this presents is that some
of the best projects for answering this question – the restoration of Cook's
cannon and the restoration of Batavia or silver coins from Western
Australia are not current. The best approach here
would be to ignore the implication of current (since it is not in the
syllabus) and talk about
any Australian projects. Restoration of an iron cannon (either from Cook's Endeavour
or the Pandora) is an obvious choice. The second choice
could be restoration of silver coins (from Dutch wrecks off
Western Australia) or restoration of wood (such as from the Batavia).
Note that the question is compare and also note the with
reference to the chemistry applied part.
State the two projects you are going to consider at the start of
your answer.
Before you can compare the techniques you need to describe what
they are. A reasonable assumption for this question is that there
will be two marks for describing the techniques in each project
and three marks for comparing them. Hence you need to keep the
descriptions brief – just enough to be the basis for the
comparison, and make the comparison as thorough as you can.
Three comparisons would seem desirable: the method of conservation
(coating with wax), the method of chloride removal (electrolysis
versus simple leaching) and the method of restoring lost or
corroded material (electrolysis for iron, wax for cellulose).
There is not too much chemistry in the restoration of wood so you
have to make the most of what there is. Therefore give the
structure of polyethylene glycol and mention how viscosity changes
with chain length.
Instead of these
projects you could have used restoration of silver coins or of a copper or brass
artefact, or perhaps of a lead one.
(CCHSC pages
433-41)
|
The two chosen projects
are the restoration of an iron cannon recovered from the Endeavour
off the North Queensland coast and the restoration of part of the
wooden hull of the Batavia recovered from off the Western
Australian coast.
The cannon was restored first by carefully chipping off coral and
limestone encrustations (no chemistry involved) then chloride was
removed by electrolysis by making the cannon the cathode at which
the reaction was
Fe(OH)Cl(s) + 2e–
® Fe(s) + OH–
+ Cl–
An inert anode was used at which:
4OH–
® O2(g)
+ 2H2O + 4e–
The electrolyte was a solution of sodium hydroxide which was
regularly replaced as chloride concentration built up. This
electrolysis also restored much of the corroded iron back to the
metal.
For its conservation, after careful washing and thorough drying
the cannon was coated with a protective wax.
For the Batavia's hull the very
fragile pieces of salvaged timber were gently washed with water to
remove sand and silt. Salvaged wood is very fragile because much
of the cellulose of wood has been eaten away by marine organisms
with only the lignin left. What remains is heavily saturated with
salt. This salt is removed by placing the wood in a solution of
polyethylene glycol in water. The polyethylene glycol gradually
displaces the salt solution. The structure of this polymer is
–(CH2–CH2–O)n–
The repeated occurrence of polar O atoms makes these polymers
water soluble. Polyethylene glycol polymers vary from watery
liquids to viscous liquids to waxes as the chain length increases
(that is as n increases).
As the salt is leached out, the polymer used is changed to a more
and more viscous one until finally a waxy one is used. This fills
up all the spaces in the wood and restores strength to the wood.
The wood is then carefully dried and coated with a wax to conserve
it.
The conservation method is the same for both artefacts – coating
it with a film of a non-reactive wax.
The method of removing chloride is different in the two cases. In
the cannon because much of the chloride is present as an insoluble
Fe(OH)Cl which cannot be leached out, electrolysis is needed to
convert the insoluble compound to soluble chloride which can be
removed. In the wood the chloride is present in soluble form and
so can be leached out over time. In the cannon much of the
corroded iron is restored back to iron metal by the electrolysis.
In the wood it is not possible to restore the wood to its original
form (structure) and so substitution of polyethylene glycol for
the missing cellulose has to be used.
|
Answers
to the 2005 and 2006 Shipwrecks Corrosion and Conservation option questions are given in
the complete Answers to HSC exam papers which you can access from the CCHSC
home page (Click on it to go there).
Answers to
exercises
1. |
Galvani
and Volta demonstrated that electricity could flow, that is, they introduced the idea
of current electricity as opposed to static electricity. Davy showed that
electricity could bring about chemical reaction: this implied that there
was some 'electrical charge' nature for at least some compounds; that is,
that some compounds are made or broken up by moving electrical charges
from one atom to another. (Much later George Stoney discovered that this
electrical charge being transferred was the electron.) Faraday showed that
there was a quantitative relation between the quantity of electricity that
passed through a circuit and the amount of chemical reaction that it
brought about; that is, that there must be a fixed amount of charge per
valency unit of the atom for changing it from neutral atom to charged
species (or ion as we now call it). It was on the basis of these ideas of
connecting chemical structure and reactivity to charged particles that
Arrhenius was able to propose his theory of ionic solutions – that
they consisted of positive and negative species he called ions. Later
scientists saw the connection between oxidation and reduction, which had
been defined in terms of gain or loss of oxygen or hydrogen or change in
valence, to electron transfer – that oxidation corresponded to loss of
electrons while reduction corresponded to gain of electrons. See
CCHSC pages 398–401.
|
2. |
(a)
|
(i) |
The value in Table
12.1 refers to an oxygen pressure of oxygen of 20 kPa. If the oxygen
pressure was 100 kPa then the solubility would be
5 x 2.8 x 10–4 = 1.4 x 10–3 mol/L
(since solubility is proportional to pressure).
Because the water
at this depth is not saturated with oxygen: the surface water could be
saturated, giving a concentration of 2.8 x 10–4 mol/L. Actually the concentration of oxygen
would decrease because bacteria in the water would use it up: hence the
low value of 1.1 x 10–4 mol/L |
|
(ii) |
The equation in
the previous section gives a value of 2.0 x 10–3 mol/L but this is for
4oC. Solubility decreases as pressure increases so a value of 1.4 x
10–3 mol/L is consistent with this. |
(b) |
1.6 x
10–2 mol/L |
|
(c) |
Because
the water at this depth is not saturated with oxygen. If
mixing took this water to a depth of 70 m, the oxygen concentration would
not increase due to the increased pressure because there would be no
source of oxygen at this increased pressure.In fact the rate of diffusion
of dissolved oxygen from saturated surface water down to this depth is
slow and much of what does diffuse down is consumed by marine organisms.
This means that the concentration at this depth is much less than the
solubility at that pressure. (CCHSC pages 427-8)
|
3. |
No;
there is insufficient moisture on the surface of the car to allow movement
of ions away from the magnesium or towards the iron for this form of
galvanic protection to work. Unless any magnesium ions formed on the Mg
block are able to move well away from the block, the tendency of Mg to
give up electrons to iron will be cancelled out.
|
4. |
Because
gold does not corrode: it is too unreactive. Surface coatings on gold are
therefore just deposits or growths that stick on to the surface rather
than incorporating some of the metal in them. Gentle abrasion generally
removes them. Electrolysis is ineffective because there is no gold
compound to reduce back to gold.
260213
|
|